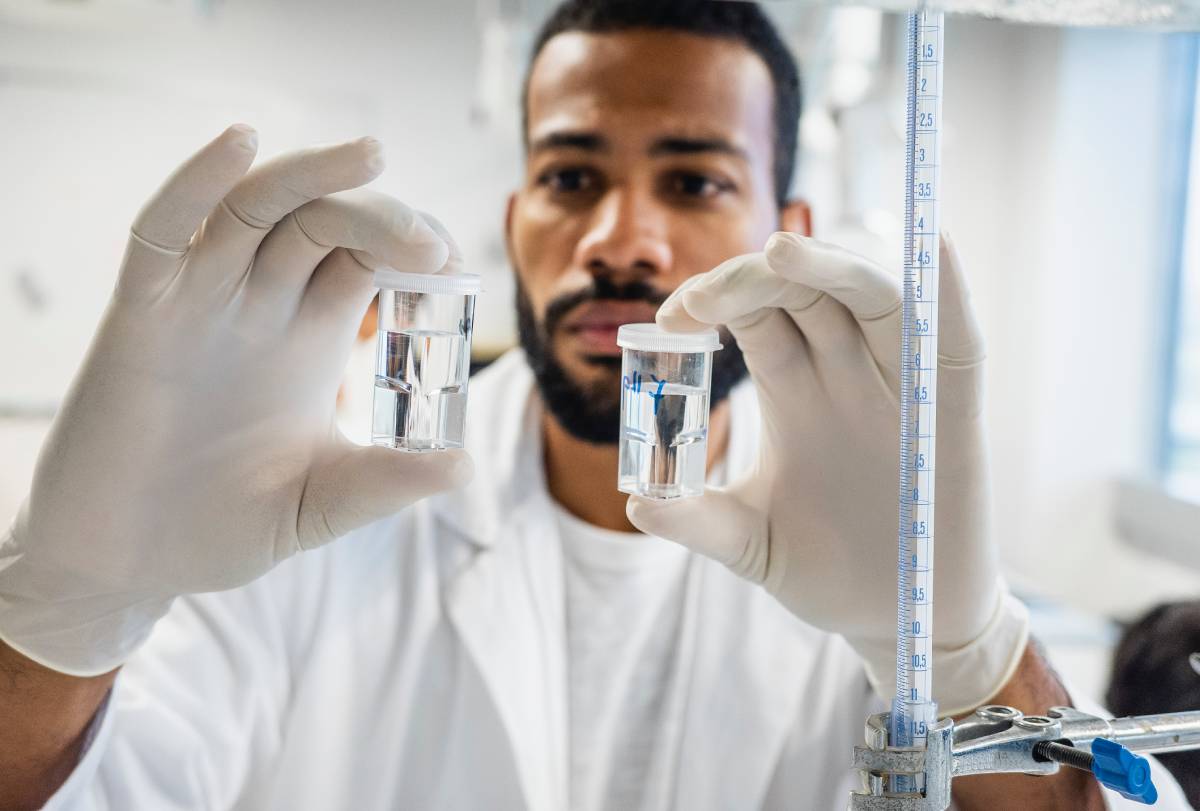
While modern anesthesia is very safe, anesthesia adverse events, or complications from anesthesia unrelated to a patient’s underlying medical condition, remain. These can include nausea, vomiting, confusion, neurotoxicity, and respiratory complications, all of which can worsen patient outcomes.1 In order to predict how a patient might respond to anesthesia, or to infer what predisposed a patient to an adverse event after the fact, researchers look to biomarkers—any measurable substance or molecule that is indicative of a disease or health outcome. Recently, researchers have identified several promising biomarkers for various anesthesia adverse events, including the few that are outlined below.
Emergence agitation (EA), sometimes known as emergence delirium, is a state of restlessness, disorientation, and incoherence that can set in as a patient wakes up from general anesthesia.2 It can lead to respiratory and circulatory problems, in addition to physical injury to patients and their caregivers.3 A team of researchers from China aimed to identify biomarkers significantly altered in the blood of patients who experienced EA.3 They found a total of 12 biomarkers significantly elevated in the EA patient group, most of which are involved in chemical reactions of fatty acids and lipids. For example, one of the biomarkers, a molecule called decanoylcarnitine, is involved in a host of essential physiological processes, including some that take place in the brain. These biomarkers, the researchers suggest, could underlie the pathology of EA and potentially serve as targets for treatments aiming to reduce the incidence of anesthesia adverse events.
In some cases, biomarkers can indicate that anesthesia may not to blame for adverse postoperative outcomes. Researchers conducted a meta-analysis of trials studying biomarkers of inflammation caused by surgery.4 The biomarkers included the immune system proteins IL6, IL10, and tumor necrosis factor alpha and were measured in over 1,600 patients of various ages and surgery types across more than 20 trials. They found that although inflammatory biomarker levels rise following surgery, there was no difference in inflammation depending on whether propofol or sevoflurane was used. This indicates that the type of anesthesia may not have influenced inflammation.
It is important to note that biomarkers can be substances or signals other than the levels of a certain molecule in the blood. A May 2024 publication in the journal Anesthesiology5 described how researchers measured patients undergoing general anesthesia using an electroencephalogram (EEG), a test that captures electrical activity in the brain, in an effort to see if there might be any observable differences in those who experienced postoperative delirium, a significant change in behavior or cognition typically considered more severe than EA.6 Of the 151 patients evaluated, all of whom were 70 years or older, 50 patients, or 33%, developed postoperative delirium. EEGs were performed the day before surgery (baseline) and as anesthesia-induced unconsciousness set in. On average, compared with the baseline, patients with postoperative delirium had lower intensity alpha waves—a type of brainwave associated with a relaxed state—while on anesthesia during the operative phase. This could indicate that EEG biomarkers obtained at the beginning of anesthesia induction could enable the early identification of patients at risk of developing postoperative delirium.
References
- Steadman, J., Catalani, B., Sharp, C. & Cooper, L. Life-threatening perioperative anesthetic complications: major issues surrounding perioperative morbidity and mortality. Trauma Surg. Acute Care Open 2, e000113 (2017), DOI: 10.1136/tsaco-2017-000113
- Lee, S.-J. & Sung, T.-Y. Emergence agitation: current knowledge and unresolved questions. Korean J. Anesthesiol. 73, 471 (2020), DOI: 10.4097/kja.20097
- Mi, X. et al. Identification of Serum Biomarkers Associated With Emergence Agitation After General Anesthesia in Adult Patients: A Metabolomics Analysis. Front. Med. 9, 828867 (2022), DOI: 10.3389/fmed.2022.828867
- O’Bryan, L. J. et al. Inflammatory Biomarker Levels After Propofol or Sevoflurane Anesthesia: A Meta-analysis. Anesth. Analg. 134, 69 (2022), DOI: 10.1213/ANE.0000000000005671
- Pollak, M. et al. Electroencephalogram Biomarkers from Anesthesia Induction to Identify Vulnerable Patients at Risk for Postoperative Delirium. Anesthesiology 140, 979–989 (2024), DOI: 10.1097/ALN.0000000000004929
- Menser, C. & Smith, H. Emergence Agitation and Delirium: Considerations for Epidemiology and Routine Monitoring in Pediatric Patients. Local Reg. Anesth. 13, 73 (2020), DOI: 10.2147/LRA.S181459