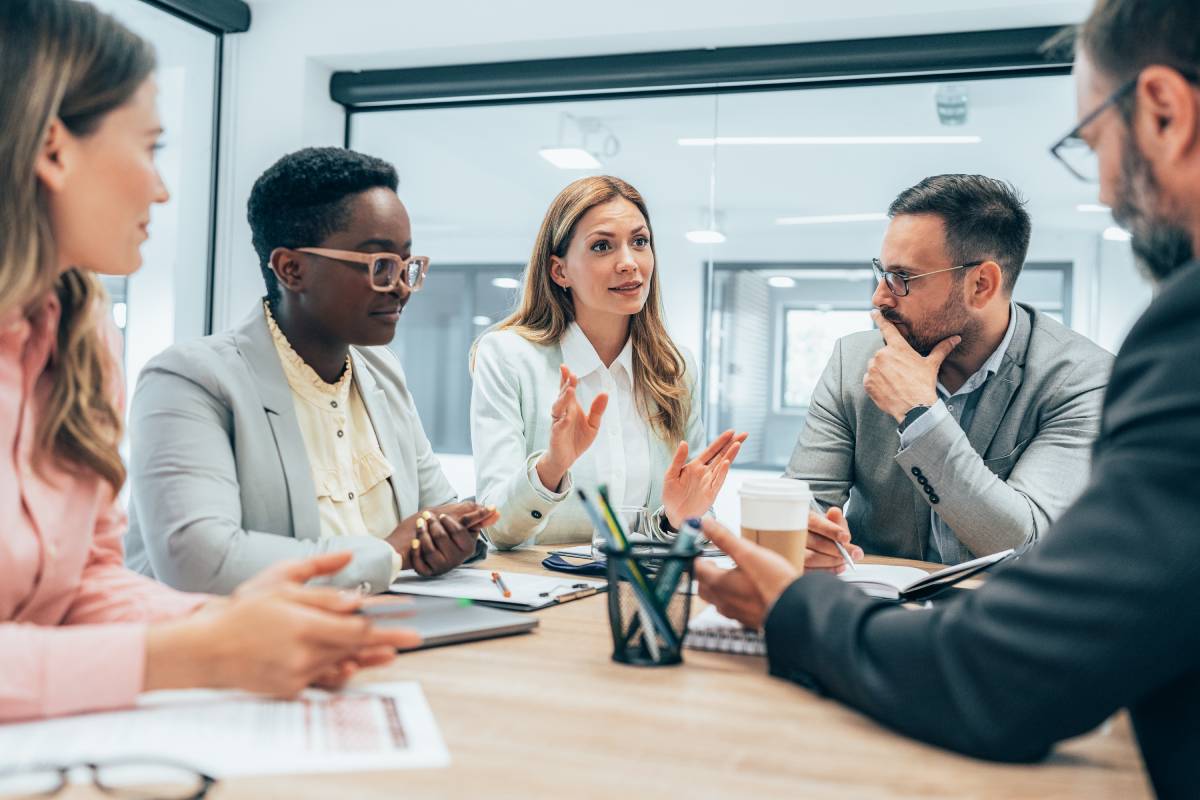
Monopolies in healthcare are a growing topic of discussion, especially within sectors like pharmaceutical companies or insurance providers. In the last year, the United States Justice Department has filed multiple antitrust lawsuits against healthcare entities (Wallace, 2024). Recently, concerns about monopolies anesthesia services have grown (Whoriskey, 2023).
Anesthesia plays a crucial role in healthcare by ensuring patient comfort and safety during medical procedures and by managing substance use and pain. However, some have expressed concerns that the provision of anesthesia services has increasingly fallen under the control of large corporate entities such as U.S. Anesthesia Partners, Inc. (USAP) (Wallace, 2024). These entities typically emerge from a series of mergers and acquisitions. USAP, for instance, originated from a large private equity firm, initiating its operations by first acquiring the largest anesthesiology group in Denver (Whoriskey, 2023). It is also now the largest provider of anesthesia services in Texas (“FTC Challenges Private Equity Firm’s Scheme to Suppress Competition in Anesthesiology Practices across Texas”, 2023).
A primary concern associated with monopolies in anesthesia—and in any industry—is the potential for diminished competition and increased pricing. When one entity dominates the market, it wields significant pricing power, which can result in increased healthcare costs for patients. For instance, USAP raised prices for some services by almost 30% during its inaugural year (Whoriskey, 2023). This escalation can further impede access to care, prompt physicians to depart from a practice, and exacerbate existing healthcare disparities. Moreover, physicians leaving monopolistic practices may face substantial financial penalties and geographic restrictions on practicing due to non-competes (Whoriskey, 2023). Notably, the Federal Trade Commission sued USAP and its founding firm in 2023, alleging a multi-year, multi-part plan to consolidate the anesthesiology market in Texas (“FTC Challenges Private Equity Firm’s Scheme to Suppress Competition in Anesthesiology Practices across Texas”, 2023). USAP rates with major insurance companies notably surpass industry averages (Whoriskey, 2023).
Another concern revolves around potential compromises in patient care. Some argue that when a single entity monopolizes the anesthesia services of a region, it may prioritize profit over patient outcomes (La Forgia et al., 2022). It is imperative to have diverse and experienced anesthesia providers who are committed to innovation while prioritizing patient welfare to continue improving the quality of healthcare (Whoriskey, 2023).
Concerns about monopolies in anesthesia, as illustrated by the USAP controversy, reflect broader concerns about healthcare costs and quality of care. Calls for enhanced transparency, oversight, and regulation within the anesthesia industry have amplified, with stakeholders advocating for measures to promote fair competition, safeguard patient interests, and ensure the delivery of quality care. Continued scrutiny of powerful healthcare systems and insurance companies is also necessary. By fostering competition, enhancing transparency, and prioritizing patient care, the healthcare industry can progress towards a more equitable and sustainable future.
References
- “FTC Challenges Private Equity Firm’s Scheme to Suppress Competition in Anesthesiology Practices across Texas.” Federal Trade Commission, Federal Trade Commission, 21 Sept. 2023, www.ftc.gov/news-events/news/press-releases/2023/09/ftc-challenges-private-equity-firms-scheme-suppress-competition-anesthesiology-practices-across.
- La Forgia, Ambar et al. “Association of Physician Management Companies and Private Equity Investment With Commercial Health Care Prices Paid to Anesthesia Practitioners.” JAMA internal medicine vol. 182,4 (2022): 396-404. doi:10.1001/jamainternmed.2022.0004
- Wallace, Claire. “Doubling down on Healthcare Monopolies: 4 Cases to Know.” Becker’s ASC Review, Becker’s Healthcare, 5 Mar. 2024, www.beckersasc.com/asc-news/doubling-down-on-healthcare-monopolies-4-cases-to-know.html.
- Whoriskey, Peter. “Financiers Bought up Anesthesia Practices, Then Raised Prices.” The Washington Post, 29 June 2023, www.washingtonpost.com/business/2023/06/29/private-equity-medical-practices-raise-prices/.