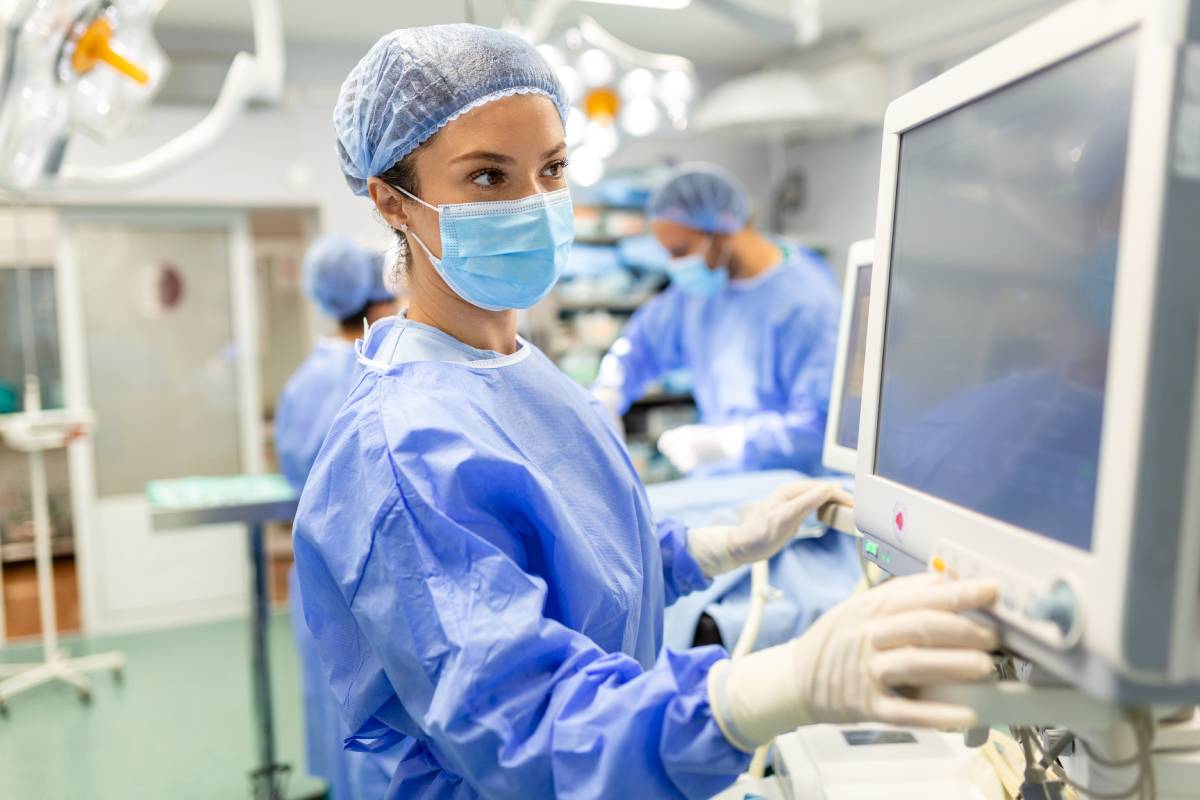
Surgical procedures are notoriously physiologically challenging: from the moment the patient goes under anesthesia, they incur a certain risk. The amount of risk, however, depends on a number of variables, including the invasiveness of the procedure, the patient’s pre-existing conditions and comorbidities, and – the focus of this article – the length of time spent under general anesthesia. Prolonged anesthesia is associated with significant risks.
Surgical anesthesia induces a host of physiological changes which would not otherwise occur in healthy patients. The chief purposes of anesthesia are to provide analgesia (pain relief) and induce loss of consciousness; however, a number of other alterations occur. It has been well documented that anesthesia can have a significant impact on blood pressure, which can in turn lead to increased heart rate and tachycardia.1 Alternatively, some drugs block baroreceptor function to inhibit this automatic feedback mechanism, allowing blood pressure to drop significantly.1 Anesthesia-induced vasodilation and alterations to the autonomic thermoregulatory response reduce the efficiency of heat conservation; a 2017 study done in China reported an incidence rate of 44 percent for intraoperative hypothermia (2). Respiratory alterations are similarly common: mainly, a reduction in tidal volume and an overall increase in respiratory rate (3). Cognitive and digestive functions become heavily impaired or suppressed, albeit temporarily (1). These accumulative changes, as well as many others not mentioned, contribute to the number of side effects associated with general anesthesia: mainly, nausea, memory loss, chills, uncontrollable shivering, urinary issues, and dizziness (4). More serious side effects – such as delirium (5) or severe cardiac events (6) – have been reported in more vulnerable populations. Side effects and risks tend to be more prevalent after prolonged instances of anesthesia.
Advancements in surgical techniques have led to an increased incorporation of minimally invasive techniques, which are more technically challenging and thus have led to increased anesthesia duration. While it might be intuitive that prolonged anesthesia would only increase patient exposure to these physiological changes and thus, increase the severity of the associated risks, the full extent of this risk was not well understood until the last two decades. For example, a 2008 landmark study from the Mayo Clinic was one of the first well-circulated papers to establish a clear relationship between postoperative complications and duration of anesthesia during surgery (7). The authors analyzed a total of 2,196 patients who had open radical nephrectomy or nephron-sparing surgery at the Mayo Clinic between 1989 and 2002. The patients were organized into three groups: those whose surgeries were shorter than 4 hours, those whose surgeries lasted 4-6 hours, and those whose surgeries lasted 6 or more hours. They found that the incidence rate of non-urological complications increased from 3.1, to 5.8, to 13.5 percent, respectively. The authors also noted a significant difference in outcomes for the patients whose surgeries lasted less than six hours compared to those whose surgeries were longer. Their findings suggested that there might be a certain “threshold” at which point anesthesia duration can significantly increase the risk of adverse effects.
Since the publication of the aforementioned study, others have demonstrated a robust association between anesthesia time and adverse effects, including venous thromboembolism, increased length of stay, and additional surgery (8). It remains unclear which exact mechanisms underlie the jump in anesthesia-associated risk when duration is prolonged to around the six-hour mark. Regardless, many medical institutions and/or private practices maintain policies regarding maximum time allowable under anesthesia, particularly for elective or low-stakes procedures.
References
1 Katzung, B., Trevor, A., & Masters, S. (2018). Basic & Clinical Pharmacology. McGraw Hill Medical Publishing Division.
2 Yi, J., Lei, Y., Xu, S., Si, Y., Li, S., Xia, Z., Shi, Y., Gu, X., Yu, J., Xu, G., Gu, E., Yu, Y., Chen, Y., Jia, H., Wang, Y., Wang, X., Chai, X., Jin, X., Chen, J., Xu, M., … Huang, Y. (2017). Intraoperative hypothermia and its clinical outcomes in patients undergoing general anesthesia: National study in China. PloS one, 12(6), e0177221. https://doi.org/10.1371/journal.pone.0177221
3 Mills G. H. (2018). Respiratory complications of anaesthesia. Anaesthesia, 73 Suppl 1, 25–33. https://doi.org/10.1111/anae.14137
4 NHS. (n.d.). General anaesthesia. NHS choices. Retrieved October 3, 2022, from https://www.nhs.uk/conditions/general-anaesthesia/
5 Evered, L. A., Chan, M., Han, R., Chu, M., Cheng, B. P., Scott, D. A., Pryor, K. O., Sessler, D. I., Veselis, R., Frampton, C., Sumner, M., Ayeni, A., Myles, P. S., Campbell, D., Leslie, K., & Short, T. G. (2021). Anaesthetic depth and delirium after major surgery: a randomised clinical trial. British journal of anaesthesia, 127(5), 704–712. https://doi.org/10.1016/j.bja.2021.07.021
6 Barker, S. J., Gamel, D. M., & Tremper, K. K. (1987). Cardiovascular effects of anesthesia and operation. Critical care clinics, 3(2), 251–268.
7 Routh, J. C., Bacon, D. R., Leibovich, B. C., Zincke, H., Blute, M. L., & Frank, I. (2008). How long is too long? The effect of the duration of anaesthesia on the incidence of non-urological complications after surgery. BJU international, 102(3), 301–304. https://doi.org/10.1111/j.1464-410X.2008.07663.x
8Phan, K., Kim, J. S., Kim, J. H., Somani, S., Di’Capua, J., Dowdell, J. E., & Cho, S. K. (2017). Anesthesia Duration as an Independent Risk Factor for Early Postoperative Complications in Adults Undergoing Elective ACDF. Global spine journal, 7(8), 727–734. https://doi.org/10.1177/2192568217701105