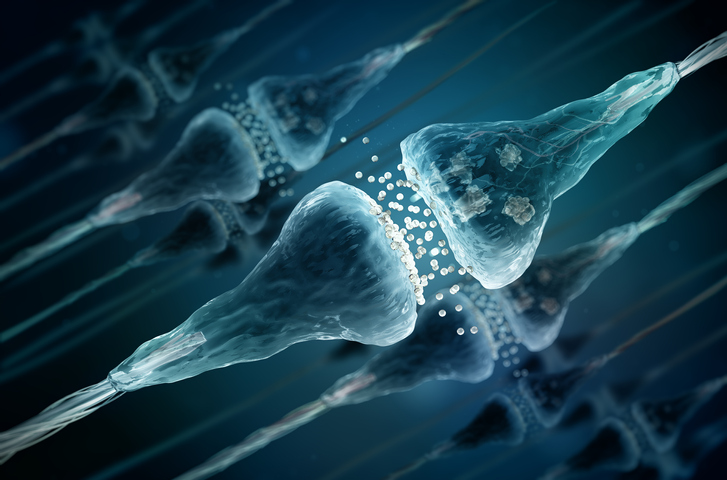
Studies on the mechanism of peripheral nerve block focus on the interaction of local anesthetics with voltage-gated sodium channels.1 Part of a superfamily of tetrameric transmembrane glycoproteins, sodium channels initiate and propagate action potentials in axons, dendrites, and muscle tissue.2-3 Sodium channels exist in three conformations: open, inactivated, and resting. During a neural impulse, the membrane potential becomes less negative as Na+ conductivity increases. At threshold, sodium channels “open” and the membrane potential shoots up.3 After a few milliseconds, sodium channels “inactivate” and the membrane potential returns to baseline.3 Once the membrane repolarizes, sodium channels return to their “resting” conformation.1 As is soon described, sodium channel conformation plays a key role in the functioning of local anesthetics.
As early as 1959, Taylor confirmed that local anesthetics selectively bind and inhibit sodium channels in nerves.4 Subsequent research localized the drug-binding site to the inner pore of the sodium channel, where local anesthetics primarily interact with a phenylalanine in domain IV S6.5 According to Fozzard et al, the affinity of local anesthetics for the binding site depends upon the conformation of the sodium channel. When the sodium channel is in its closed state, the binding affinity is low and Na+ current is only blocked at high concentrations (mM range).5 In contrast, the binding affinity is much higher in the open and open-inactivated states, during which the voltage sensors are deployed outward.5
Strichartz was first to observe the frequency-dependence of local anesthetics.6 According to the model, local anesthetic inhibition of Na+ current increases with repetitive depolarizations.1 Statistically, the percentage of sodium channels in the open and open-inactivated states at any given moment increases as the frequency of action potentials inceases.1 Since the binding affinity is higher for the open state relative to the resting state, a lower concentration of anesthetics is therefore needed at higher frequencies.1
Although the biochemistry at the binding site is well understood, it is not fully understood how local anesthetics block Na+ current at the biophysical level. One possible mechanism is that local anesthetics obstruct the inner pore of sodium channels. Molecular modeling from Hanck et al is consistent with the idea that local anesthetics bind horizontally across the pore.7 According to Sunami et al, the high-affinity binding site is about 10 Å below the selectivity filter, where charged ligands block the channel by occupying sites NaIII or NaIV for permeant ions.2,7 Even if the channel is not completely obstructed, the positive charge placed at the innate sites creates an electrostatic barrier that blocks Na+ influx by Coulombic repulsion.2 Therefore, local anesthetics block Na+ current by a combination of physical and electrostatic obstruction.
An alternative explanation is that local anesthetics interfere with gating. In this model, local anesthetics reduce the movement of charged residues in the voltage sensor, or gating current.5 More specifically, Fozzard et al found that the binding of local anesthetics to phenylalanine locks domain III S4 in its outward position. This prevents the voltage sensor from resetting during repolarization, thereby disrupting the gating mechanism of sodium channels and blocking Na+ current.5
In summary, local anesthetics bind to a phenylalanine in domain IV S6 of sodium channels. The affinity of local anesthetics for this binding site is highest when the sodium channel is in the open state. Once at the binding site, local anesthetics create a physical and electrostatic barrier that blocks Na+ current. In addition, local anesthetics may also interfere with the gating mechanism of sodium channels. Further research is still needed to clarify the biophysical mechanisms by which local anesthetics block Na+ current.
References:
1) Becker, Daniel, and Reed, Kenneth. “Local anesthetics: review of pharmacological considerations.” Anesthesia progress vol. 59, 2 (2012): 90-101.
2) “Clinical Pharmacology of Local Anesthetics.” New York School of Regional Anesthesia. 2019.
3) Vadhanan, Prasanna et al. “Physiological and pharmacologic aspects of peripheral nerve blocks.” Journal of anaesthesiology, clinical pharmacology vol. 31, 3 (2015): 384-93.
4) Taylor, RE. “Effect of procaine on electrical properties of squid axon membrane.” Am J Physiol vol. 196 (1959): 1070–1078.
5) Fozzard, Harry et al. “The sodium channel as a target for local anesthetic drugs.” Frontiers in pharmacology vol. 2, 68. 1 Nov. 2011.
6) Strichartz, GR. Local Anesthetics: Handbook of Experimental Pharmacology. Springer-Verlag, 1987.
7) Sunami, A, Dudley, SC, and Fozzard, HA (1997). “Sodium channel selectivity filter regulates antiarrhythmic drug binding.” Proc. Natl. Acad. Sci. U.S.A.